1. From point measurement to systems understanding
The ideal: an instrument that can conduct non-destructive tests to determine the entire elemental and molecular composition and dynamics of a living system, process or material as well as the chemical, physical and biological interactions at submicrometre level and on microsecond time scales.
We can already measure many phenomena, either with advanced instruments in the lab or with sensors out in the field. However, we cannot answer such obvious questions as ‘How much microplastic is in my pizza and what does this imply for my health?’, ‘Is the fine dust in the air I’m breathing right now hazardous to my health?’, ‘What is magnitude of reactive-nitrogen deposition here?’ or ‘What kind of plastics is this and how can I recycle it best?’, even if we know the precise composition of the food or air in question. That is because we cannot fathom complex reality at a systems level. We can acquire the necessary understanding by considering material reality and its dynamics from different perspectives, and by studying how the whole system of components behaves. This requires complementary and, often, dissimilar measurements from different perspectives, at different levels of physical, chemical or biological detail, and at different length and time scales. These measurements must also be carried out in a way that does not influence the system.
There are a number of ways to improve our understanding of systems. First of all, we must increase the level of detail of our measurements. We should also develop non-invasive and non-destructive monitoring methods. We must further develop, use and combine complementary techniques that analyse differing aspects of the object of study and its internal dynamics. Finally, we must develop generic methods for combining entirely different types of output, information and knowledge about complex systems or their elements.
2. Measuring where and when necessary
The ideal: In the future we will have a sensor for personalised food; a factory that adapts its production parameters continuously based on the latest composition of raw materials; a system that measures plastics and metal recycle streams en indicates how it can best pe processed for optimal value retention; a camera for immediate and specific detection of harmful viruses and sensors for continuous and localized measurement of substances and their levels in water and air.
Detecting abnormalities as soon as they occur allows us to take precautions even without understanding the entire system. This, however, requires having enough data available and knowing what a significant or worrisome abnormality is. In the case of individuals, a sign of good health in one person may indicate a worrying turn of events for another.
To save time and make rapid intervention possible, we have to be able to measure, process the output and, in many cases, arrange feedback on and transport of the results at the location of measurement. In fact, the laboratory should be brought to the object or person being measured, or even be inserted into them. The miniaturisation of high-tech instruments is therefore crucial. Because untrained users will increasingly be in charge of the measurements, extra demands will need to be made on robustness and effectiveness. Validation and standardisation are vital in this respect. It is important for users to have the result presented as reliably and unambiguously as possible. The questions ‘What should we measure?’ ‘How, where and how often should we measure?’ ‘Who should be getting feedback?’ and ‘Can we combine different measurements or results?’ call for communication between researchers in the relevant areas of application, in analytical science, in standardisation, and in design and systems integration.
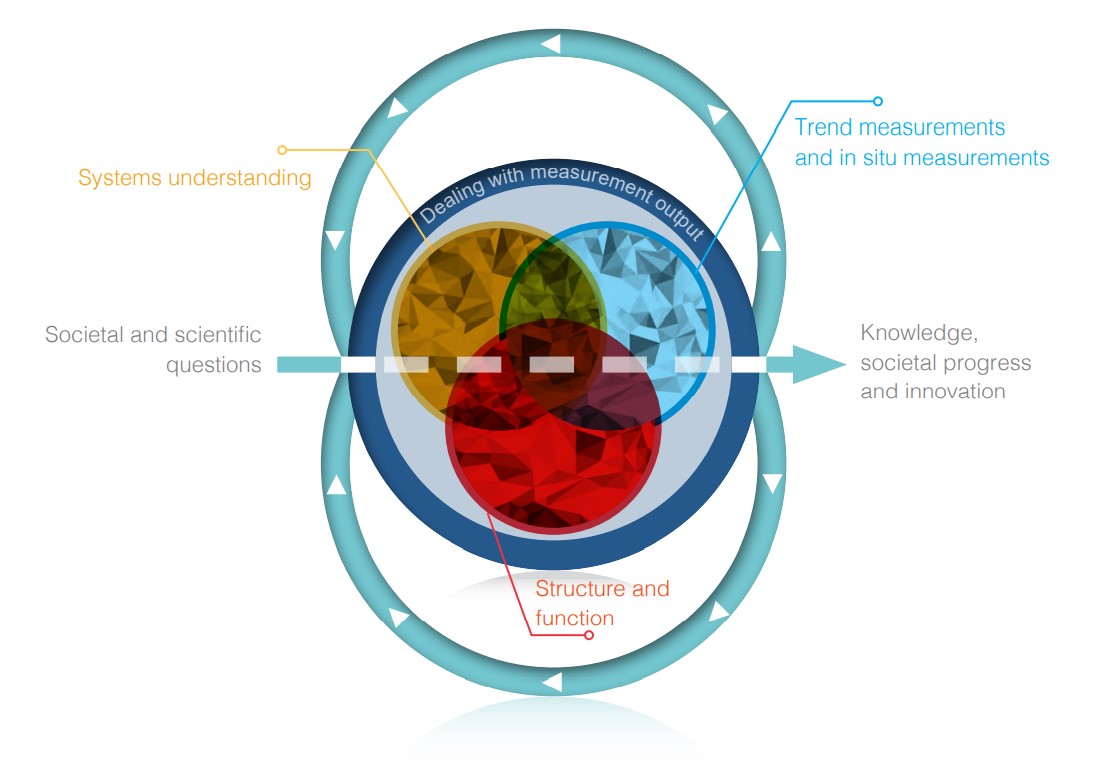
3. Understanding the relationship between structure, composition and function
The ideal: An expert system that can predict the possible conformations of a chemical substance under variable circumstances based on structural information; an expert system that indicates how substances of very high concern in products can be replaced by safe alternatives, and an interdisciplinary platform for studying structure/property and structure/performance relationships.
The key to understanding systems is to comprehend the function or behaviour of the separate components of that system and how they interact with one another. This is of eminent importance in pharmacology, where we need to understand both the effects and side effects of drugs or identify toxicity levels without animal testing. The first task is to get a thorough understanding of a substance by applying multiple techniques for structural analysis and by studying it under varying conditions. This type of practical research can be enhanced by chemical and physical conformational calculations.
The second step is to determine the compound’s functional performance. In essence, this involves developing ‘structure/performance relationships’. One key success factor is to have instruments for studying the behaviour of matter and the dynamics of its components while in use, without disrupting the system. There are a few examples in science and industry in which structure and property or performance are linked, but these are relatively limited and there is no generic scientific model available. Dutch public-private partnerships in the relevant fields would support the development of a platform for structure/performance relationships.
4. Learning to deal with output
The ideal: To move from analytical research to an analytical society; to link physics, chemistry, biology, statistics, ethics, law, psychology and design with growers, environmental experts, nutritionists, patients, physicians, civilians, operators and other users, so that scientists and innovators know which users they serve and that users know what for and how to use the results.
In only five years’ time, we will be using more than 250 billion sensors worldwide in all sorts of applications, from monitoring the environment to monitoring patients, from monitoring plants to measuring the quality of the built environment. This is a factor of our society’s growing need for certainty and risk avoidance, for example in terms of mental and physical health, nutrition, safety, and materials integrity. People will not only 'live in a sensorium’ but become part of the team that measures and interprets the output.
Untrained users will thus gain access to miniaturised equipment, with the risk that they will use it incorrectly at times. This trend will give rise to a growing need for validated and standardised methods to determine and guarantee the quality of the results.
Because such output will be used without expert intervention, for example in the Internet of Things, a new form of quality assurance is needed. While it can be based in part on existing quality assurance methods and techniques, a large dose of innovation will also be required. Education can also help both laypersons and researchers to avoid inaccurate measurements and the incorrect use of output. It is vital to know the strengths and weaknesses of the various methods, as well as the relevant levels of accuracy, selectivity, specificity and a good understanding of the aim and use of the measurements. Training individuals in the basics of measurement is not the problem; the challenge lies in adapting the research to allow us to identify the most effective didactic methods. This calls for experts in instrument engineering, statistics and didactics to join forces and embark on joint research.
By improving our understanding of systems, science will no doubt be able to reduce the margin of uncertainty, but uncertainty is inherent to measurement. We must make it clear to users what margins of uncertainty actually mean. This is especially relevant when the measurement or monitoring results comprise bad news. That is why research with links to psychology is needed to study public perception and the best way to present results. The aforementioned link to didactics also plays a role here.
Sensors will not only tell us a lot about life and matter, but also about environments and even individuals. Progress in instrumentation will also give rise to discussions about intellectual property, public acceptance and privacy. Who owns the output data, the information and the knowledge, and what can and can’t we do with it? What can we do with incidental discoveries? The answer may well lie in unique combinations of ethical and legal research, but not before we estimate the potential impact and risks of the data falling into ‘the wrong hands’. Security experts must be involved to develop risk management solutions. Researchers must also consider how to raise public awareness of the value of measurement results.
It is more than obvious that we need to set up a multidisciplinary platform focusing on the social, ethical and legal aspects of measurement and detection. An entirely new field, analytical logic, must be launched and coordinated through existing alliances in analytical science and technology and the other relevant disciplines mentioned above.
(Updated route text 2023)
A pdf of the Dutch version of the route description can be found here.